by Daniel Gregory
University of California, Riverside, CA, USA
The Earth has undergone profound changes in the chemistry of its oceans and atmosphere from the time before life began to the present day. This has resulted in a wide variety of different ocean and atmospheric compositions, including variations in oxygen composition, over the last 3 billion or more years of Earth’s history. Understanding what these changes were has important implications for not only a better understanding how life came into being, but also to predict what atmospheres may nurture life on planets that are orbiting other stars.
Conventional ways to determine the chemical conditions of oceans at a given time involves getting samples of rock that formed during that time period, analyzing the chemical constituents of the rock, and interpreting the results. However, the earth is a dynamic environment and rocks are often buried so deep that their chemistry gets altered, which makes whole rock analyses difficult, if not impossible, to interpret. Luckily some components of sedimentary rocks are more resistant to these processes than others. One such component is the mineral pyrite — otherwise known as fool’s gold.
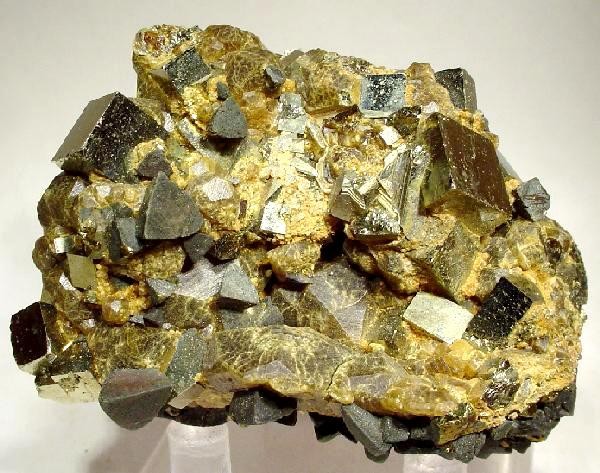
Pyrite, also known as “fools gold.” Rob Lavinsky, iRocks.com – [CC BY-SA 3.0], via Wikimedia Commons
A technique called “laser ablation inductively coupled mass spectrometry” (LA-ICPMS) uses a laser to vaporize a small amount of pyrite. That vapor is then passed through a machine called a “mass spectrometer” to determine what elements are in the vapor. To test this relatively new technique, we went to a well-known geologic section of the Doushantuo Formation in southern China that is composed of a specific sequence of siltstones. Siltstone is a type of sedimentary rock made of layers of silt. This section of rock has been used for a lot of different studies that used conventional whole rock analyses to determine the chemistry of the oceans during a rise in oxygen during the second half of the Neoproterozic Era (720-541 million years ago). Previous studies identified three different intervals of enrichment of oxygen-sensitive trace elements (like molybdenum, vanadium, uranium, and rhenium) and interpreted them to represent periods of increased oxygen.
To prove that pyrite trace element chemistry can also be used to make the same interpretations of oxygen levels, we analyzed pyrite from the same samples used in these earlier studies. We found that several elements (molybdenum, silver, antimony, selenium, lead, cadmium, and tellurium) are enriched at the same time periods as found by the conventional techniques, indicating increased oxygen levels in the atmosphere.
The reason for this is at times of lower oxygen abundance, there is more H2S on the seafloor, and because there is more H2S, more pyrite forms. Trace elements are drawn down to the seafloor in greater abundance over a wider area when oxygen is low. This results in lower trace elements in the ocean and less trace elements in pyrite. Conversely, when there is more oxygen, less of the ocean floor is sulfidic, so less pyrite forms. That leads to higher levels of trace elements in the ocean and also in pyrite where it is still being formed. Additionally, higher oxygen levels may have also led to an increase in oxidative weathering of rocks on land, which would have contributed additional trace elements to the oceans.
This enrichment of trace elements in pyrite and traditional whole rock studies show that pyrite trace element chemistry is successful in tracking the same pulses of oxygen as traditional techniques and further supports its ongoing use in paleo-oxygenation studies. This is a major game changer for paleo-ocean chemistry studies because a common problem with old rocks is that they are altered and cannot be used in traditional whole rock studies. Pyrite, on the other hand, preserves its original trace element content through most geological processes. So, analyzing pyrite gives us a glimpse into the ocean’s history and lets us measure past ocean oxygen conditions better than traditional methods. This will allow better interpretations of the atmospheres in the Earth’s past, which can then be used to decipher whether life may have existed under atmospheres we can measure on exoplanets.